In this article, I discuss a quantity that is extremely well predicted and measured, and that subsequently offers a great handle to constrain new phenomena in particle physics. This quantity is named the anomalous magnetic moment of the muon.
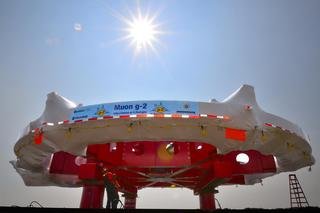
[image credits: Fermilab]
It is extremely precisely predicted by the Standard Model of particle physics, the value being
0.00116591828 ± 0.00000000049
On the other side, the best measurement (measured using the magnet in the picture) is given by
0.00116592089 ± 0.00000000063
Again: precision.
There is however only one chance out of about 1000 that these two results agree, when error bars are accounted for. Oho?! Isn’t it where new phenomena could occur?
In this post, I will define what is the anomalous magnetic moment of the muon and explain how those very precise predictions and measurements are made and can be used to constrain new phenomena in particle physics.
MUONS
In the anomalous magnetic moment of the muon, there is the word muon. I know I have defined those beasts in other posts, but I would like to try to write something self-contained today.

[image credits: the particle zoo]
A muon is the little guy represented by a plush on the left (particles can always be represented by plushes).
More seriously, a muon can be seen as an electron, but in a heavier version. It has the same electric charge, spin and properties.
One exception, the muon is unstable so that after some time, it decays into an electron and two invisible neutrinos.
Muons have been discovered (in the 1930s) thanks to the fact that Earth is bombarded by muons, originating from cosmic rays, on a regular basis. Thousands of them indeed reach each place on Earth every minute.
Muons are deeply studied since then, the measurement of their anomalous moment being one of the most famous study.
MAGNETIC MOMENTS
Let us focus on the title of the post another time and the magnetic moment part in particular.
The magnetic moment of an object tells us about the magnitude and direction of the rotational force that it feels when it is plunged into an external magnetic field.

[image credits: Pixabay]
To take a simple example, we can imagine a compass.
The needle of the compass will rotate to align itself parallelly to the surrounding magnetic field.
The rotational force (or the torque) experienced by the needle is proportional, on the one hand, to the magnetic field (the more intense is the field, the larger is the torque) but also, on the other hand, to the magnetic moment.
But the relative direction between both also plays a role, so that when both the magnetic moment and the magnetic field are aligned, the torque vanishes and the needle stops.
We actually say that the magnetic moment of any object tries to get aligned with the surrounding magnetic field.
WHERE ARE MAGNETIC MOMENTS COMING FROM?
There are two contributions to a magnetic moment (that must be summed): a first comes from the motion of electric charges, and a second is related to the intrinsic nature of the object constituent.
For instance, the magnetic moment of a hydrogen atom is given by the sum of the intrinsic moment of the constituting proton and electron, as well as the contribution stemming from the motion of the electron around the proton.
This intrinsic magnetic moment (μ) is the one we are interested in. It is determined by the spin (S), the mass (m) and the electric charge (q) of the particle under consideration,
μ = gs q/2m S
But what is this gs-factor in the middle of the formula? This quantity is actually called the g-factor, and it can be predicted by the theory. The predictions say g=2.
THE ANOMALOUS MAGNETIC MOMENT OF THE MUON
However, the microscopic world is quantum… and quantum mechanics implies that there are quantum corrections to the value of the g-factor, making it slightly different from 2. The difference with 2 is called the anomalous magnetic moment.
One can calculate those quantum corrections, in the context of the Standard Model of particle physics, and one gets the number shown at the beginning of this post:
0.00116591828 ± 0.00000000049

[image credits: RCNP]
Experimentally, muon beams are prepared by first smashing protons on a fixed target, and by then collecting the remnants of these collisions. After a while, those remnants (called pions) decay into muons that are gathered into a beam.
The experimental value has been measured at the Brookhaven National Laboratory,
0.00116592089 ± 0.00000000063

[image credits: BNL]
To handle this measurement, physicists prepared a muon beam, switched on a huge magnetic field and studied the behavior of the muons in the field.
The spin of the latter precesses, and the precession rate can be very precisely measured.
From the related measurements (through the observation of the properties of the muon decay products) and a very good knowledge of the magnetic field, physicists can then extract the experimental value of the anomalous magnetic moment of the muon with a very good precision.
TOWARDS NEW PHENOMENA
There is thus a slight difference between the theoretical and experimental value of the anomalous magnetic moment of the muon.
The reason may be that the deviation between theory and experiment stems from some new particles that we have not observed yet, and that must thus be included in the calculations**.
Inverting the argument, we have here a way to constrain the presence of new particles indirectly. They should preferably contribute in reducing the gap between theory and experiment (or there are chances that the new theory can go to the bin).
SUMMARY AND REFERENCES
In this post, I discussed a little bit what is the anomalous moment of the muon. I detailed how the theoretical predictions and the experimental measurement are made.
Although new measurement will come (data taking at the g-2 experiment at Fermilab has just started), I quickly sketched how current results can already be used as constraints on new phenomena.
As a bonus and for a little reward: where is the name “g-2” for the Fermilab experiment coming from?
References:
- The topic of this post was inspired by this article that I have recently read.
- More information on magnetic moments can be found on many lecture notes available all over the web, and even on Wikipedia.
- Experimental information can be found on the BNL website (an older experiment) here and on the Fermilab website (the new experiment) there.
For more discussion on this topic (or anything related to science), please join us on #steemSTEM. The steemSTEM project is a community-supported project aiming to increase the quality and the visibility of STEM (STEM is the acronym for Science, Technology, Engineering and Mathematics) articles on Steemit. You can also follow our curation trail on Streemian to support us.
I can't wait to hear & learn so much more about this stuff!
P.S. "G" has a slightly higher value than 2, therefore the name given to the experiment was "g minus 2" (g-2)
Looking forward to some good discussions on the future of physics :-)
Finally someone who answers my question! It seems I was waiting for such a comment forever :D
[You will get some upvotes for your comment, as promised.]
What a shame that you had to wait for so long...wait no more my friend :-)
Indeed :)
th upvoter of your post, I get a great number. :PVery good knowledge @lemouth, I am the 100
Peace, Abundance, and Liberty Network (PALnet) Discord Channel. It's a completely public and open space to all members of the Steemit community who voluntarily choose to be there.Congratulations! This post has been upvoted from the communal account, @minnowsupport, by Ecomp from the Minnow Support Project. It's a witness project run by aggroed, ausbitbank, teamsteem, theprophet0, someguy123, neoxian, followbtcnews/crimsonclad, and netuoso. The goal is to help Steemit grow by supporting Minnows and creating a social network. Please find us in the
Wow...that is great article thank very much prof. Very complete teory. I like new information about scien
I do my best :)
Nice article.
I am not an expert in quantum, but it was really nice to read about muons and chances of the presence of new unknown particles due to difference in theoretical and experimental values.
Actually this is how science advances. From theories we get idea of new experiments and from experiments we get new theories. Both are complementary, and that's why this is science. :D
The level of calculation is such that even when you know how it works, it is tough to go to such a level of precision. Only a few people around the world can.
Great post,really enjoyed reading it and extremely interesting even though it's a bit advanced for me,i've never even heard of a muon before.Can't believe the miniscule measurements that we are able to measure it's absoulely amazing.thanks for sharing.
Building the experimental detector and experiments is also a interesting and not easy challenge. It took more than a couple of brains to achieve that :)
Hello @lemouth... The science of physics that studies with magnet .. Maybe you yourself are familiar with this magnetic object because many found in various tools of everyday life. Utilization of magnets in everyday life is very much but often not realized because the layman just use the tool only and do not recognize what components are in it. Good post friends ..
Thanks for your message! We can even say that electromagnetism is in general everywhere.
Great post...about science..
Thanks for Sharing, you are all amazing !
This post has received a 2.90 % upvote from @buildawhale thanks to: @trumpman. Send 0.100 or more SBD to @buildawhale with a post link in the memo field to bid on the next vote.
Steem WitnessTo support our curation initiative, please vote on my owner, @themarkymark, as a