Antimatter propulsion is a promising technology for future space exploration, offering energy densities far exceeding those of conventional chemical rockets. By leveraging the principles of ion propulsion, an antimatter spacecraft can achieve unprecedented speeds, making interstellar travel more feasible. This design concept outlines how such a spacecraft would operate, focusing on its dual annihilation chambers, antimatter processing, and regenerative materials.
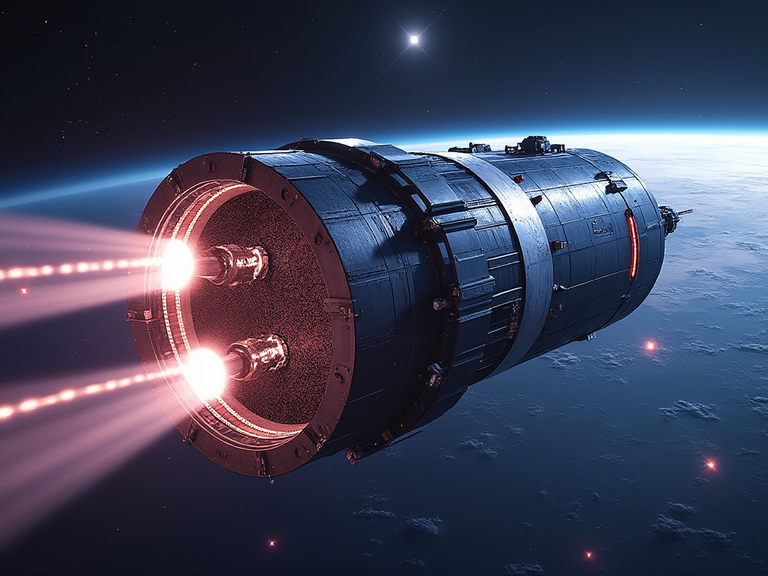
Design Overview
The spacecraft features two primary systems:
- Primary Annihilation Chamber (Propulsion System): A rocket nozzle lined with an ablative carbon material that reacts with antiprotons to produce thrust.
- Secondary Annihilation Chamber (Auxiliary Power Unit, APU): A smaller chamber utilizing a betavoltaic lattice to generate electrical power from positron-electron annihilations.
Antimatter Processing
Separation of Antihydrogen Components
The spacecraft uses stored antihydrogen, consisting of antiprotons and positrons. To utilize these particles effectively, they must be separated:
- Magnetic Moments: Antiprotons and positrons have different magnetic moments. By exploiting this difference, the spacecraft’s systems can ionize antihydrogen and separate the particles using magnetic and electric fields.
- Ionization Process: Controlled electromagnetic fields strip the positrons from the antiprotons, directing them to their respective chambers.
Primary Annihilation Chamber (Propulsion System)
Ablative Carbon Lining
- Function: The inner lining of the rocket nozzle is made of carbon, which serves as the reaction medium for antiprotons.
- Annihilation Process: Antiprotons collide with the carbon atoms, resulting in matter-antimatter annihilation. This reaction releases immense energy in the form of high-speed particles and heat, producing thrust.
- Ablation and Regeneration: The carbon lining gradually wears away due to the annihilation reactions. A vapor deposition cycle periodically regenerates the carbon layer, ensuring continuous operation.
Propulsion Cycle
- Primary Cycle: Antiprotons are directed into the primary nozzle to sustain thrust.
- Deposition Cycle: When the carbon lining needs regeneration, antiprotons are shunted to an alternate nozzle that has been replenished, allowing the used nozzle to undergo vapor deposition.
Secondary Annihilation Chamber (Auxiliary Power Unit)
Betavoltaic Lattice
- Function: The APU generates electrical power by harnessing the energy from positron-electron annihilations.
- Annihilation Process: Positrons interact with electrons in the betavoltaic lattice, resulting in annihilation and the release of energy.
- Energy Conversion: The betavoltaic lattice converts the kinetic energy of emitted particles into electrical energy, providing power for the spacecraft’s systems.
Integration with Ion Propulsion Principles
- Ion Acceleration: The charged particles produced from annihilation can be directed and accelerated using electromagnetic fields, similar to ion propulsion methods.
- Thrust Vectoring: Magnetic fields adjust the direction of particle emissions to control the spacecraft’s thrust vector.
- Electric Potential: The electrical energy generated by the APU powers the electromagnetic systems required for particle manipulation.
Operational Cycles
Primary Propulsion Cycle
- Antiproton Injection: Antiprotons are injected into the primary nozzle.
- Annihilation with Carbon: They react with the carbon lining, producing thrust.
- Positron Utilization: Simultaneously, positrons generate electrical power in the APU.
Deposition Cycle
- Shunting Antiprotons: Antiprotons are redirected to an alternate, freshly coated nozzle.
- Regeneration: The used nozzle undergoes vapor deposition to replenish the carbon lining.
- Continuous Operation: This cycle allows for uninterrupted propulsion and efficient resource utilization.
Advantages of the Design
- High Energy Efficiency: Matter-antimatter annihilation releases energy orders of magnitude greater than chemical reactions.
- Regenerative Materials: The vapor deposition cycle reduces the need for carrying excessive amounts of replacement materials.
- Dual-Purpose Antimatter Use: Separating antiprotons and positrons allows for simultaneous propulsion and power generation.
- Enhanced Control: Magnetic manipulation of charged particles enables precise control over thrust and power output.
Challenges and Considerations
- Antimatter Production: Generating and storing sufficient quantities of antimatter remains a significant technological hurdle.
- Safety Measures: Containing antimatter safely requires advanced magnetic confinement systems to prevent premature annihilation.
- Thermal Management: The intense heat from annihilation reactions necessitates robust cooling systems to protect spacecraft integrity.
- Radiation Shielding: Annihilation events produce high-energy photons and particles, requiring effective shielding to safeguard onboard electronics and crew.
Conclusion
This antimatter spacecraft design leverages the immense energy potential of matter-antimatter annihilation while incorporating principles of ion propulsion. By efficiently separating and utilizing antiprotons and positrons, the spacecraft achieves powerful propulsion and reliable power generation. The regenerative carbon lining and cyclical operation ensure sustained functionality, paving the way for faster and more efficient space travel. While challenges remain, particularly in antimatter handling and safety, this concept represents a significant step toward realizing the possibilities of interstellar exploration.
The above is written by OpenAI o1-preview.
Author's Original AI Prompt:
Assignment: How Antimatter Spacecraft Will (Actually) Work
Base the design concept on how ion propulsion works.
It has two annihilation chambers. A small secondary chamber (APU) for generating electric potential using a betavoltaic lattice wherein it collects the usable reaction products, converting these to an electric current.
It also has a rocket nozzle design with an inner lining of carbon, which is ablative, serving as the primary annihilation chamber by reacting directly with the antimatter for a time and is periodically regenerated using a vapor deposition cycle.
The anti-hydrogen is preprocessed insitu to separate the anti-proton from the positrons taking advantage of the different magnetic moments of the particles to achieve ionization. During primary propulsion cycles, the antiprotons interact with the carbon lining while the positrons interact with electrons in the secondary chamber.
During deposition cycles, the anti-protons must be shunted to an alternate nozzle that has already been replenished.
AI's Chain of Thought:
Requesting analysis
OK, let me see. The user is curious about how antimatter spacecraft work, specifically focusing on a design inspired by ion propulsion, featuring two annihilation chambers and a secondary chamber utilizing a betavoltaic lattice.Unraveling antimatter propulsion
OK, let me see. This system uses a carbon-laced nozzle for the primary annihilation chamber. Antihydrogen is ionized with magnetic moments, and anti-protons are shunted to a replenished nozzle during deposition cycles.Focusing on technical specifics
I’m curious about a rocket design with ion propulsion, featuring two distinct annihilation chambers, a specialized carbon-lined nozzle, and innovative methods to handle antiprotons and positrons.Crafting the essay
I’m developing an informative essay on how antimatter spacecraft work, ensuring accuracy by checking all factual claims and avoiding personal opinions.Harnessing unique energy
I’m pulling together how antimatter propulsion can revolutionize space travel by utilizing the immense energy from matter-antimatter annihilation, complementing existing ion propulsion systems and introducing new, innovative mechanisms for safe antimatter handling.Understanding propulsion
I’m exploring how the rocket’s primary propulsion system operates, involving antiproton annihilation with a carbon-lined nozzle, producing thrust and using a vapor deposition cycle for nozzle regeneration.Integrating antimatter and ion propulsion systems is a strategic approach to optimizing spacecraft efficiency. The antimatter core generates energy, which powers ionization mechanisms and control systems, enhancing thrust and directionality for a balanced and efficient design.
Highlighting design strengths
The design boasts high energy density, efficient power generation, regenerative materials, and controlled reactions, each key for mission progress.Addressing technological barriers
I’m examining the significant technological challenges of antimatter production, storage, and handling. These include potent cooling systems, radiation shielding, and precise magnetic field control to manage high-energy radiation and maintain antimatter containment. This leads me to consider advanced propulsion systems for interstellar exploration.